In the first part of this article, we discussed how room-temperature photoluminescence spectroscopy can be used to characterise material properties of atomically-thing transition metal dichalcogenides (TMDs). In Part II, we will focus on experimental setups used for these measurements, describe their main components, and briefly talk about building your own micro-photoluminescence setup.
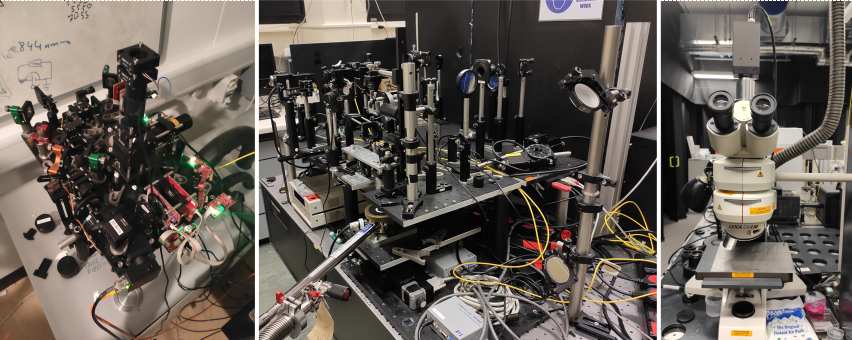
Micro-photoluminescence setups (often abbreviated to microPL or μPL) are optical setups designed to measure luminescence spectrum of samples with a very small size (down to or even below few μm) emitted under optical excitation. They can vary substantially in their structure and appearance depending on specific application of this technique (see examples in Fig.1) but will always include four main components: excitation source, optical system, detector, and sample imaging and positioning system. Let’s discuss these elements in more details.